News Story
Waging War on Metastatic Cancer
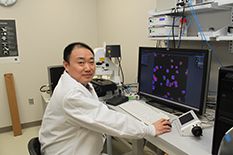
Across nearly all types of cancer, the number one cause of death is not the primary tumor itself, rather, it is the spread of cancer to other parts of the body. This spread, known as metastasis, occurs when cancer cells break away from a primary tumor site and travel via the bloodstream or the lymphatic system to form new tumors in other parts of the body.
For decades, researchers have worked to uncover new methods to slow or stop the growth of metastatic cancer. But, these efforts are limited in large part due to poor understanding of the factors that lead cancer cells to form tumors in the first place. Adding to these challenges, scientists still have much to learn about why some cancer cells prove resistant to drug therapies while others do not.
This critical juncture is exactly where Fischell Department of Bioengineering (BIOE) Professor Xiaoming (Shawn) He and his team of researchers step into the picture.
He and his team have dedicated years of tireless work to tackling two key questions: what causes cancer to metastasize, and could strategically targeted therapies halt even the fastest-moving cancers?
To address the former challenge, He and members of his lab teamed up with researchers from The Ohio State University, the University of Maryland’s Marlene and Stewart Greenebaum Comprehensive Cancer Center, and the University of Missouri School of Medicine to shed light on a prevailing theory that among the many types of cancer cells embedded within a malignant tumor are cancer cells that act as stem cells.
Cancer stem cells (CSCs) are rare; if a scientist were to examine a randomized cluster of 100 cancer cells, it’s possible that only one – or none – would feature CSC characteristics. Yet, CSCs are believed to be the main culprit in cancer relapse and metastasis. CSCs alone may regenerate and repopulate a tumor, and researchers like He believe that CSCs are often the true drivers of cancer metastasis and relapse. Even more, He believes that advanced understanding of CSCs could shed new light on why certain cancers prove unresponsive or resistant to therapies.
Think of it this way: if CSCs are the roots that drive a cancer’s growth or spread, conventional chemo and radiotherapies aimed at shrinking the whole tumor could prove fruitless in the long run. So long as the CSCs remain intact, the risk of cancer relapse or metastasis would remain.
“CSCs are more drug resistant than bulk tumor cells,” He said. “As a result, they often survive conventional chemo and radiotherapy and there’s added risk that they could enter the blood vessels cut open during a conventional surgical resection of a tumor. This could spawn cancer relapse at both local and distant locations in the body.”
Recognizing this, He and his collaborators achieved a breakthrough discovery that supports the stem cell hypothesis. Last year, the team developed a novel technique to isolate a single cancer cell that fits the proverbial mold of a CSC. Inspired by nature’s own process – whereby a single cell proliferates into a cell colony in a miniaturized nanoliter core – He and his team created a miniaturized 3D microcapsule to encapsulate one single cancer cell in such a way that they could mimic the conditions under which stem cell colonies form from a single cell in an embryo.
Unlike previous methods to study CSCs, He’s team successfully isolated CSCs in such a way that they could demonstrate what’s known as cross-tissue differentiation. This means that, for the first time, He’s group demonstrated that CSCs act like regular stem cells – they can divide and proliferate to form a host of different types of cells, such as skin cells, bone cells, or even neurons and cardiac cells.
“For years, many researchers have been reluctant to refer to these unique cells as CSCs and, instead, they have called them tumor initiating cells, or TICs,” He said. “This is because contemporary methods to isolate CSCs could not produce the necessary data to prove that CSCs carry out cross-tissue differentiation in the same way that normal stem cells do. By enabling the understanding and targeting of what we have now proven to be CSCs, our study may open a whole new world in the field of cancer biology and therapy aimed at eliminating cancer from its root.”
Even more, by effectively isolating CSCs, He’s group conducted analysis that proves what many have long believed: CSCs are highly resistant to conventional therapies, metastatic, and tumorigenic – meaning that they can survive traditional cancer treatments and go on to spread tumors to other sites in the body.
Since publishing the group’s findings in Advanced Science, He and fellow researchers have applied this work to further studies on aggressive breast cancer and drug-resistant cancers.
A Mission to Render “Drug-Resistant” Cancers Defenseless
With renewed understanding of what drives cancer to regrow and spread, He and his team can also dive deeper into the factors that enable certain cancers to develop a resistance to chemotherapy drugs, otherwise known as multidrug resistance.
One of the primary reasons cancer cells develop resistance is the overexpression of what are known as efflux pumps – proteins that protect a cell by pumping out unwanted toxic substances before they can reach their intended target. In the same way that efflux pumps work hard to protect against toxins, they also expel virtually all clinically relevant chemotherapy drugs.
Recognizing this, scientists have investigated how various kinds of nanoparticles capable of evading this process could be used to deliver anticancer drugs into multidrug-resistant cancer cells. Unfortunately, existing studies have shown that drugs can still be easily pumped out of cells once they are released from the nanoparticles, which limits the potential effectiveness of drugs delivered into multidrug-resistant cancer cells.
To combat this challenge, He and a team of researchers developed a technique for direct inhibition of the drug efflux pumps themselves. In order to do this, He’s team engineered a specialized nanoparticle that would target tumor vasculature and specifically release a drug that inhibits P-glycoprotein, the protein that enables efflux pumps to function, and anticancer drugs into tumor cells. Their findings were published this month in Nature Communications.
To date, the group’s studies show promise that their technique could be used to deliver anticancer drugs to hard-to-reach cancer cells. Even more, the group’s findings indicate that their method could potentially be used in combination with a specialized laser irradiation technique to improve the effectiveness of chemotherapy drugs.
Taking Aim at Triple-Negative Breast Cancer
In 2020, Xiaoming (Shawn) He earned a prestigious National Institutes of Health (NIH) Innovative Research in Cancer Nanotechnology (IRCN) Grant to support his efforts to target a uniquely aggressive form of breast cancer known as triple-negative breast cancer (TNBC).
About 10 to 20 percent of breast cancers are considered triple-negative, which means that, unlike most breast cancers, this particular type is not fueled by the hormones estrogen or progesterone, nor by the HER2 protein.
While treatments for most other forms of breast cancer work by targeting one of these three avenues, TNBC does not respond to modern hormonal therapies or medicines that target HER2 protein receptors. As such, most TNBC patients are limited to chemotherapy as their only systemic treatment option.
All cancers originate as the result of changes that have occurred within the genes of a cell or group of cells. In the case of triple-negative breast cancer, a gene known as TP53 is most frequently deleted or mutated.
But, TP53 is critical. It provides instructions for making a protein called p53 that helps prevent the development of tumors by stopping cells with mutated or damaged DNA from growing and dividing uncontrollably. Although many researchers have considered techniques to restore p53 activity, no such therapy has been translated into the clinic, owing to the complexity of p53 signaling.
Recognizing this, He and his research team have instead focused efforts on POLR2A – an essential neighboring gene of TP53. The group chose this route because genomic alterations tend to be large regional events in a cell. Most cancers that lead to the loss of a particular tumor suppressor gene also lead to the partial loss of nearby genes such as POLR2A, a gene that is essential for any cell to survive.
Although cancer cells can survive a partial loss of POLR2A, they become weakened and vulnerable to POLR2A inhibition. Knowing this, He and his research team hypothesized that targeted inhibition of POLR2A could potentially kill TNBC cells while sparing normal cells.
To explore this option, the team looked to RNA interference (RNAi) with small interfering RNA (siRNA), a biological process by which RNA molecules inhibit gene expression or translation. This process can be used to precisely target virtually any genes – including those that may contribute to cancer growth.
The challenge, however, is that siRNA is extremely unstable in blood and in endosomes and lysosomes, the digestive system of cells. To overcome these obstacles, He and his research group designed “nano-bomb” particles that they could use to protect POLR2A siRNA in blood circulation and carry the siRNA into the targeted tumor for cells to “eat.” The particles then generate CO2 gas to break open endosomes and lysosomes to ensure timely release of siRNA to inhibit POLR2A.
He believes that his group’s findings offer hope that one day a nanotechnology-based precision-targeting strategy could be used to fight TNBC and many other types of cancer.
###
The aforementioned research is supported by the National Cancer Institute of the National Institutes of Health under award numbers R01CA243023 and R01CA206366.
The opening portion of this article references an Advanced Science paper titled “Bioinspired One Cell Culture Isolates Highly Tumorigenic and Metastatic Cancer Stem Cells Capable of Multilineage Differentiation.” Along with He, the following contributed to the paper: first author Hai Wang (BIOE; National Center for Nanoscience and Technology, Beijing [NCNT]; the University of Chinese Academy of Sciences [CAS]), Pranay Agarwal (The Ohio State University [OSU]), Bin Jiang (BIOE), Samantha Stewart (BIOE), Xuanyou Liu (University of Missouri), Yutong Liang (BIOE), Baris Hancioglu (OSU), Amy Webb (OSU), John P. Fisher (BIOE), Zhenguo Liu (University of Missouri), Xiongbin Lu (Indiana University),and Katherine H. R. Tkaczuk (The University of Maryland’s Marlene and Stewart Greenebaum Comprehensive Cancer Center).
The research discussed in “A Mission to Render ‘Drug-Resistant’ Cancers Defenseless” was published in Nature Communications under the title “Carbon nano-onion-mediated dual targeting of P-selectin and P-glycoprotein to overcome cancer drug resistance." Along with He, the following contributed to the paper: first author Hai Wang (BIOE; National Center for Nanoscience and Technology, Beijing [NCNT]; the University of Chinese Academy of Sciences [CAS]), Yutong Liang (BIOE), Yue Yin (NCNT), Jie Zhang (NCNT), Wen Su (NCNT) Alisa White (BIOE), Bin Jiang (BIOE), Jiangsheng Xu (BIOE), Yuntian Zhang (BIOE), Samantha Stewart (BIOE), and Xiongbin Lu (Indiana University School of Medicine).
In addition to his BIOE appointment, He is a faculty member of the University of Maryland’s Robert E. Fischell Institute for Biomedical Devices, as well as the University of Maryland’s Marlene and Stewart Greenebaum Comprehensive Cancer Center.
Published January 19, 2021